Unlocking Quantum Dynamics: The Surprising Robustness of Many-Body Scars Amid Noise
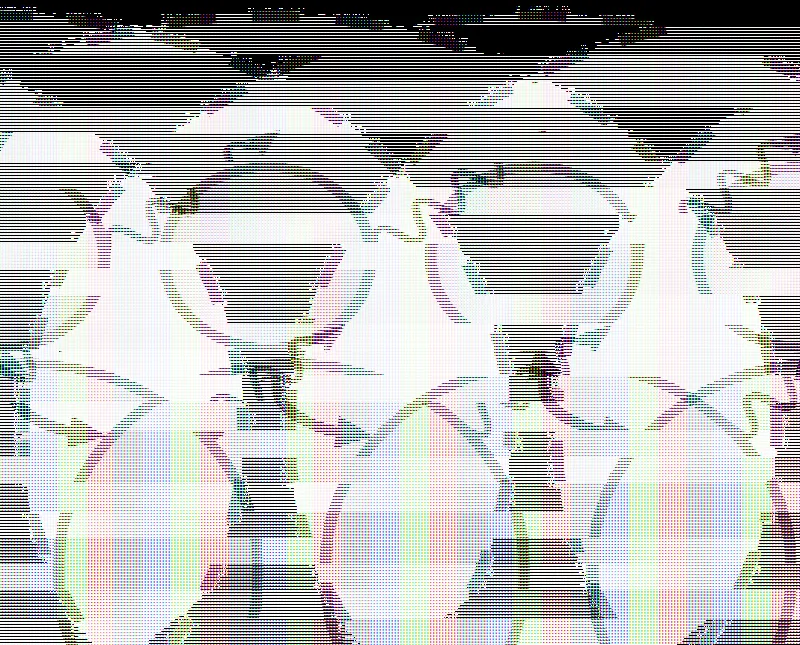
Recent breakthroughs in quantum physics reveal the surprising resilience of quantum many-body scars (QMBS) even under noisy environments. A captivating study conducted by researchers at Zhejiang University and the University of Leeds has uncovered a phenomenon referred to as the Liouvillean spectral transition, showcasing that these quantum states can withstand levels of environmental interference that were previously thought to lead to full chaos.
Understanding Quantum Many-Body Scars
Quantum many-body scars are unique states within quantum systems that can arise when the system is not entirely chaotic, which is typically expected in many-body physics. These scars exhibit non-thermal behavior and have drawn significant attention due to their potential applications in quantum computing and information processing. In this study, the researchers explored how these scars behave under pure dephasing, a common form of environmental noise that disrupts quantum coherence.
The Spectacular Spectral Transition
The core finding of the study is that as the dephasing strength increases, the scarred eigenmodes of the Liouvillean undergo a transition reminiscent of spontaneous PT-symmetry breaking. Unlike chaotic systems, which often thermalize quickly under such perturbations, the approximate QMBSs maintain their scar-like dynamics. Notably, the critical dephasing rate at which this transition occurs shows a surprisingly weak dependence on the system size, indicating a robust nature of the scars in larger quantum systems.
Robust Dynamics in Noisy Simulators
The implications of these findings are significant for the development of robust quantum technologies. By demonstrating that QMBSs can function effectively in noisy environments, the study suggests that these states could be utilized in practical quantum simulators and computers, boosting their efficiency and reliability. The research highlights specific model platforms such as the XY spin ladder and the PXP model of Rydberg atom arrays, where these scars exhibit noteworthy resilience against decoherence.
Practical Applications and Future Directions
This research not only enhances our understanding of QMBSs but also opens up avenues for exploring their utility in quantum sensing and information encoding—areas where quantum states can provide advantages over classical systems due to their intricate properties. Future investigations may focus on how different types of noise impact QMBSs and understanding the underlying mechanisms that confer such robustness, potentially guiding the design of next-generation quantum technologies.
As quantum simulations continue to evolve, insights from this study will undoubtedly play a pivotal role in shaping their development and implementation in real-world applications.